Water is the most valuable and abundant resource on the globe, covering 71% of the Earth’s surface (oceans) and providing habitat to 97% of all living things. The oceans and other saline waterways constitute the world’s greatest water supply, accounting for 97.4% of all available water. This article looks at the use of alternative water resources in copper leaching processes in the Chilean mining industry. The research was published in Metals.
The remaining water (2.5%) largely (1.7%) corresponds to polar and glacier caps, which is why it is difficult to use. Traditional sources such as lakes, groundwater, and rivers account for only 0.8% of the total.
Freshwater is necessary for all living things. A human embryo’s body is made up of 97% water, a newborn’s body includes 80% water, and an adult’s body contains 60–65% water. If only the freshwater available on the planet is considered, the vast majority (68%) is encased in ice and glaciers, while the remaining 30% is entombed.
Freshwater supplies are presently under severe strain around the world as a result of rapid population expansion, quicker economic growth in certain countries, and improvements in people’s living conditions, all of which result in increased consumption of this resource. This is compounded by the fact that water is dispersed unevenly in practically every corner of the globe.
The Middle East and North Africa (MENA) region is an example of unequal distribution and reduced availability. This area is mostly deserting, receiving less than 1% of the world’s water.
The acid leaching of copper sulfide minerals using saltwater and wastewater from water desalination facilities is the subject of this study, which includes a bibliographic analysis based on the most recent scientific articles.
The goal of this paper is to highlight the major issues and obstacles that big-scale copper mining presents when using these water resources in significant quantities. The instance of Chile will be discussed in this document because it is the world’s greatest copper producer and the country with the most projects involving the use of seawater and wastewater in mining.
Discussion
Mining consumes a modest amount of water on a global scale. Even yet, it is usually a big water consumer on a local basis. Mining is Chile’s fourth-largest water user, accounting for 3% of total consumption. Freshwater scarcity in dry zones is a financial, ecological, and social issue.
In Chile, mining consumes the majority of the country’s water (51%) in the Antofagasta region, which is situated in the Atacama Desert, the country’s driest region. Due to a lack of freshwater resources, this condition causes tensions between mining firms and local populations.
In general, continental water consumption is predicted to reach 12.5 m3/s by 2030—a drop of 6% from 2020—as illustrated in Table 1.
As indicated in Figure 1, by 2030, seawater consumption might increase by 120% compared to 2020, with seawater accounting for 47% of the water required for copper mining on a national scale.
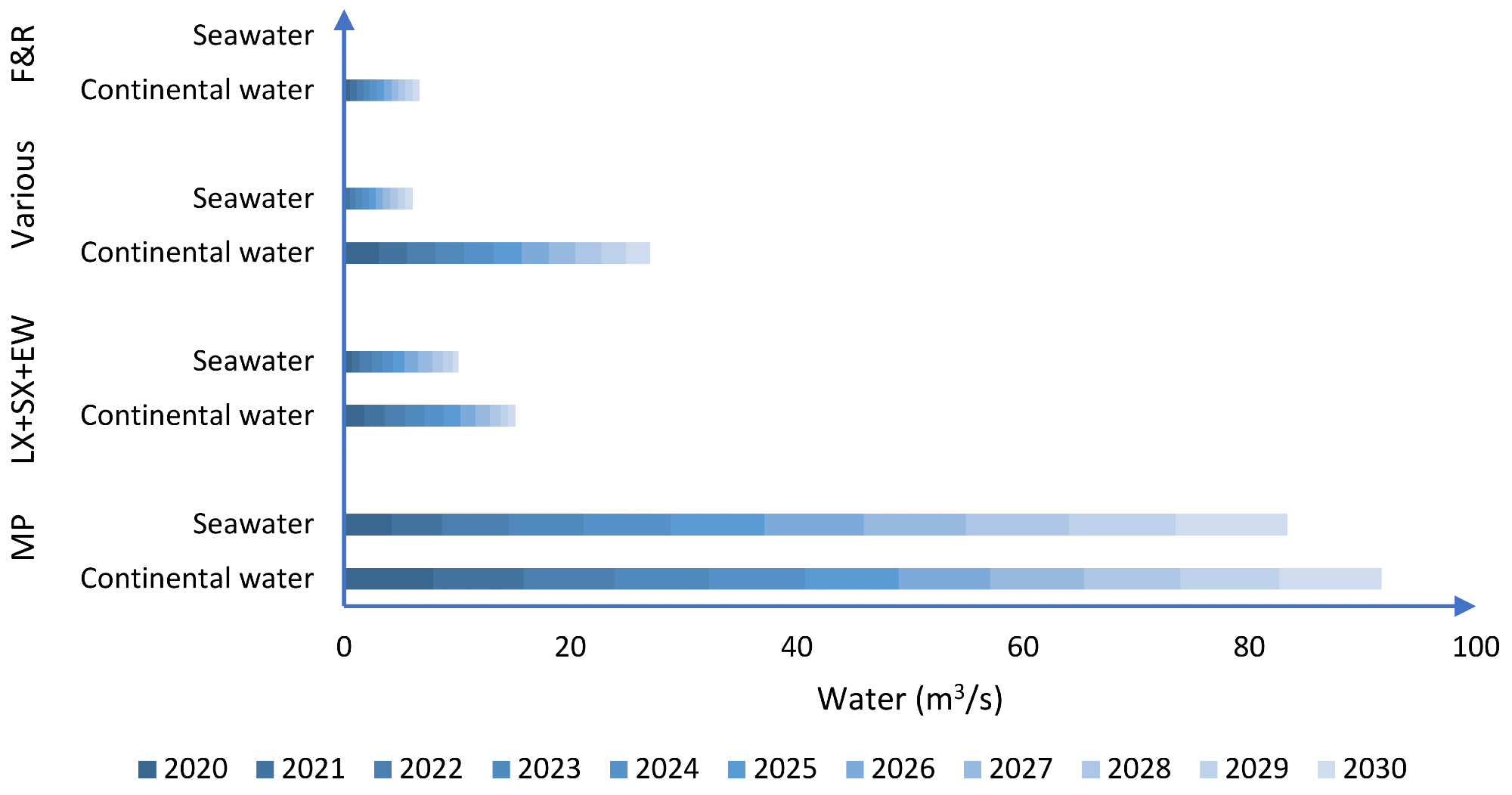
Figure 1. Distribution of water consumption according to mining process (MP: mineral processing; LX: leaching; SX: solvent extraction; EW: electrowinning; F&R: smelting and refining processes. Image Credit: Cochilco, 2015
Table 1. Projection of expected water consumption in copper mining. Source: Cochilco, 2015
Water (m3/seg) |
2020 |
2021 |
2022 |
2023 |
2024 |
2025 |
2026 |
2027 |
2028 |
2029 |
2030 |
Continental water |
13.3 |
12.8 |
12.9 |
13.2 |
13.4 |
12.9 |
12.4 |
12.6 |
12.3 |
12.3 |
12.5 |
Seawater |
5 |
5.6 |
7.5 |
8.2 |
9.2 |
9.9 |
10.6 |
10.9 |
10.7 |
10.9 |
11 |
Total |
18.3 |
18.4 |
20.4 |
21.4 |
22.6 |
22.8 |
23 |
23.5 |
23 |
23.2 |
23.5 |
Mineral concentration processes (MP) are predicted to consume 73% of continental water by 2030, followed by hydrometallurgical processes (5%), mine water and utilities (17%), and smelting and refining procedures (5%). This trend continues for saltwater, with mineral processing accounting for the majority of demand (90% by 2030) (Figure 1).
Presently, the use of seawater in manufacturing processes is a viable alternative to restricted water resources. Many mining companies are experimenting with using seawater in their operations. Some companies use it directly in their procedures, while others desalinate it before introducing it to their plants.
The steps outlined in Figure 2 are followed when using seawater in mining activities.
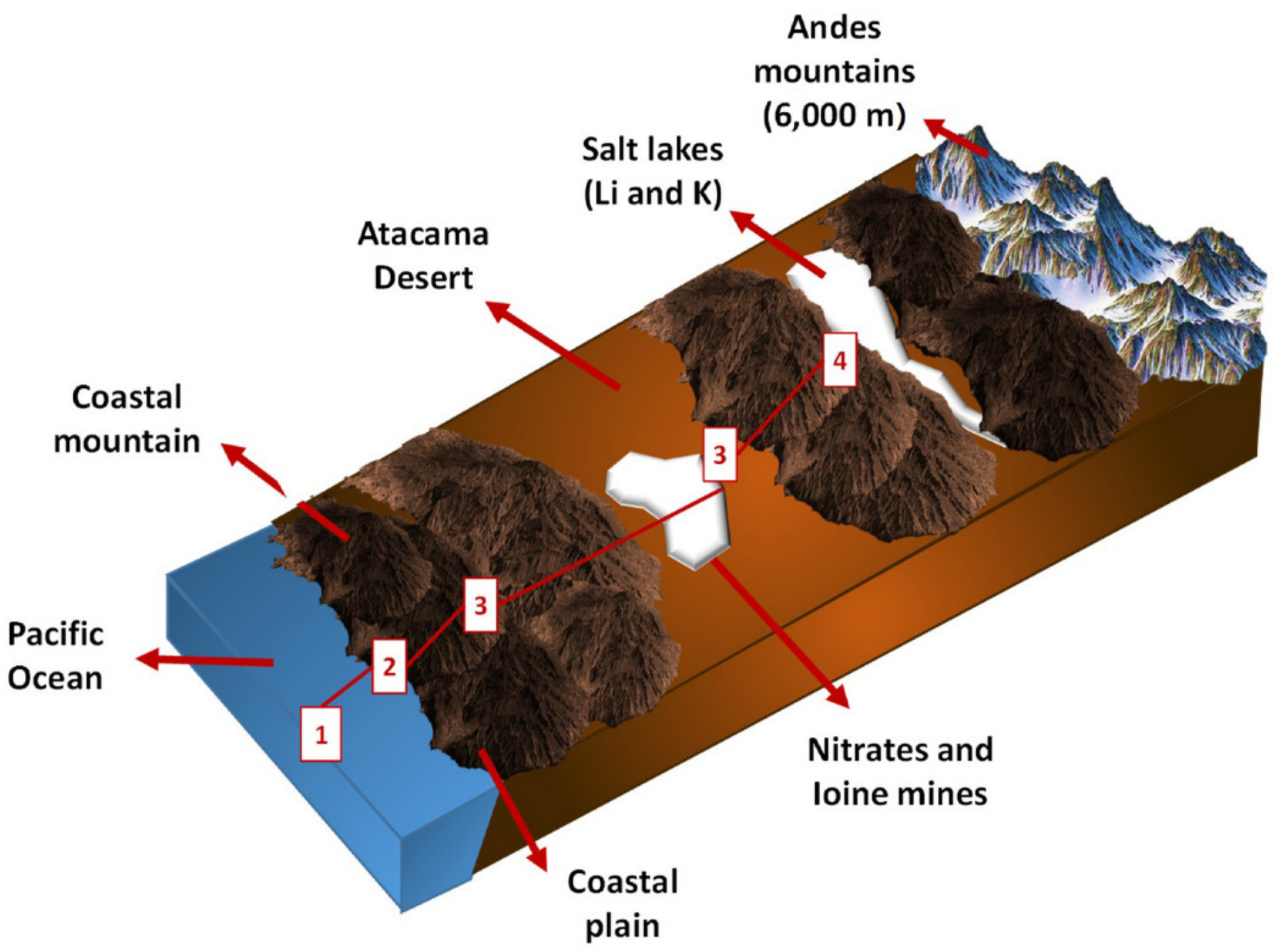
Figure 2. Geographic scheme of the Antofagasta region in Chile, mining activities and seawater collection system. Image Credit: Cisternas, & Gálvez. 2018
Various research employing salt water for the dissolution of primary copper sulfides and secondary copper sulfides have been performed for leaching procedures. In general, all research agrees that integrating seawater into leaching processes has a favorable effect. This is primarily owing to the presence of chloride ions (~20 g/L) (Table 2).
Table 2. Reference composition of seawater. Source: Toro, et al., 2020
Solute |
g/kg of Solution |
Na+ |
10.78145 |
Mg2+ |
1.28372 |
Ca2+ |
0.41208 |
K+ |
0.3991 |
Sr2+ |
0.00795 |
Cl− |
19.35271 |
SO42− |
2.71235 |
HCO3− |
0.10481 |
Br− |
0.06728 |
CO32− |
0.01434 |
B(OH)4− |
0.00795 |
F− |
0.0013 |
OH− |
0.00014 |
B(OH)3 |
0.01944 |
CO2 |
0.00042 |
Total |
35.16504 |
Due to the oxidizing potential of nitrate ions, the researchers tested the concentration of sodium nitrate in the system and found favorable results, increasing copper extraction from 27.9 to 80.2% in media based on saltwater and from 14.8 to 63.9% in media based on distilled water (Figure 3).
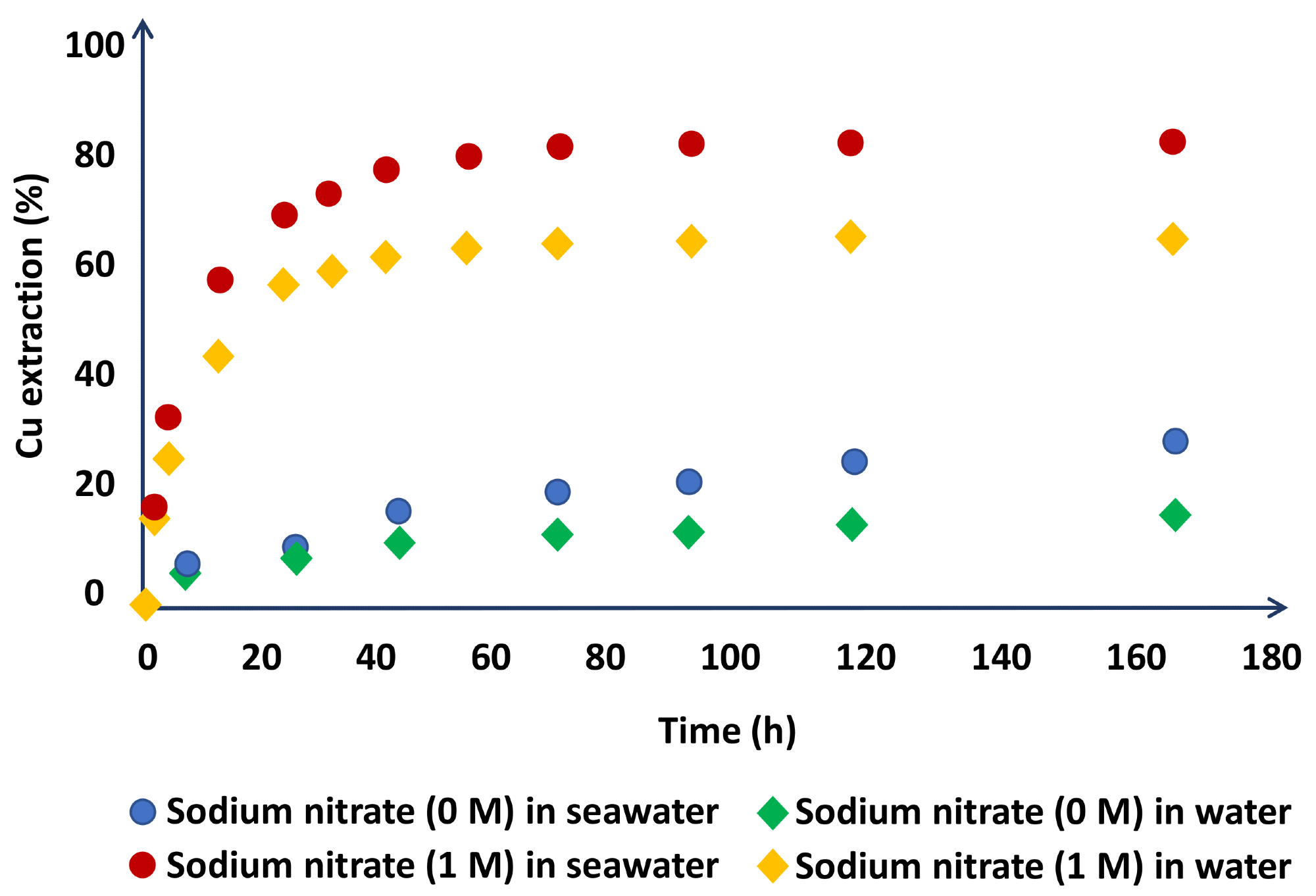
Figure 3. Chalcopyrite dissolution in the leach liquors using H2SO4 (1 M) + NaNO3 (0 or 1 M) in seawater- and water-based media (at 45 °C). Image Credit: Hernández, et al., 2018
In terms of acid concentration, Hernández et al. demonstrated that working with 0.5 M H2SO4 in seawater yields identical copper extractions to working with 1M H2SO4 in distilled water (Figure 4).
Furthermore, Castellón et al. show that ion oxidation rises with increased acidity levels in the leaching solution, owing to the generation of NOx gas over time. The creation of this gas, according to the researchers, allowed the system’s potential to be sustained at over 700 mV vs. Ag/AgCl, promoting chalcopyrite leaching (Figure 5).
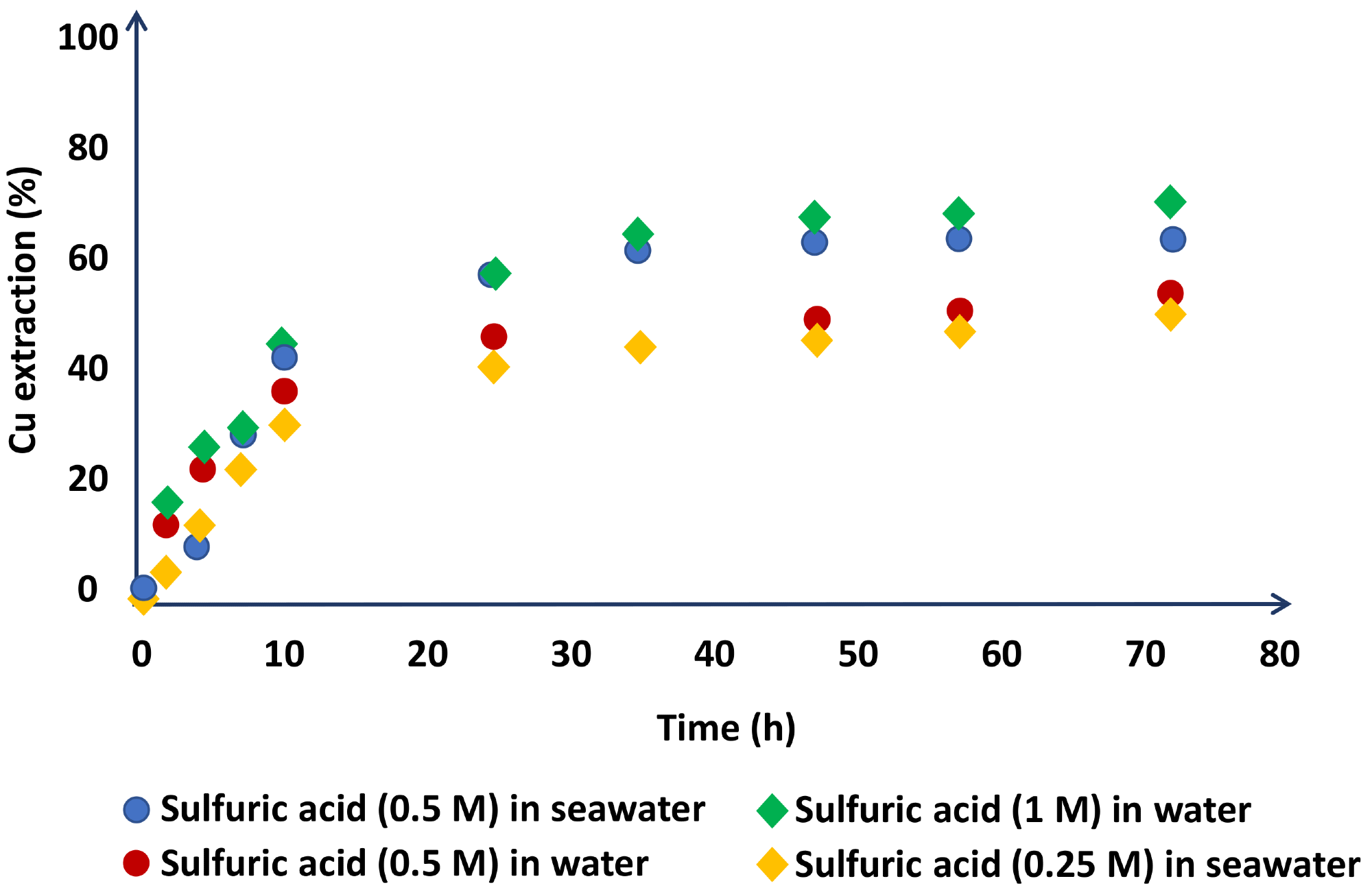
Figure 4. Chalcopyrite dissolution in the leach liquors with experimental conditions of NaNO3 (1 M), three days of leaching, 45 °C. Image Credit: Hernández, et al., 2018
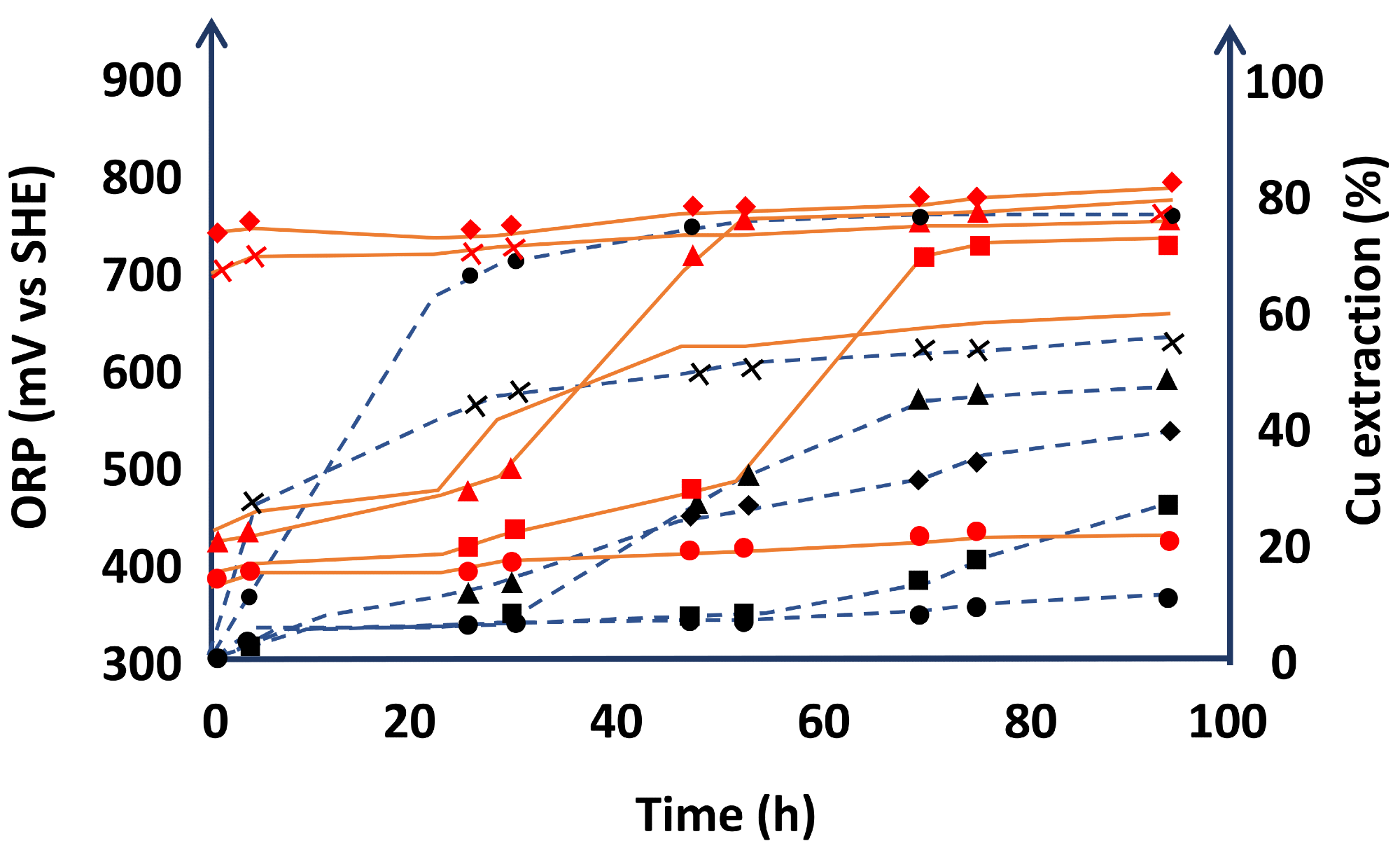
Figure 5. Dissolution of chalcopyrite concentrate and averaged ORP (mV vs. Ag/AgCl) values over time (hours) for Series I at different concentrations of H2SO4 and NaNO3. (o) Test 1 (H2SO4 = 0.1 M and NaNO3 = 0.1 M); (▪●◊∆◌•) Test 2 (H2SO4 = 0.1 M and NaNO3 = 0.5 M); (N) Test 3 (H2SO4 = 0.5 M and NaNO3 = 0.1 M); (•) Test 4 (H2SO4 = 0.5 M and NaNO3 = 0.5 M); (X) Test 5 (H2SO4 = 1.0 M and NaNO3 = 0.1 M); (_) Test 6 (H2SO4 = 0.5 M and NaNO3 = 0 M). (Test conditions: 94 h, P80 = 60.66 µm, 450 rpm and room temperature). The copper recoveries are shown with black dotted line in axis secondary and averaged ORP values are shown with red dotted line. Image Credit: Castellón, et al., 2020
Hernández et al., on the other hand, evaluated the effect of adding NaCl to the same amount of chloride as seawater in their studies for the leaching of a chalcopyrite concentrate in an acid medium using seawater and caliche salts (Figure 6a).
Furthermore, these systems have similar behavior in their potential redox values, as seen in Figure 6b. This is in line with Toro et al.'s investigations of chalcocite leaching in an acid media using seawater. When dealing with seawater and 20 g/L chloride, the researchers received comparable findings (Figure 7).
![(a) Comparison of copper extraction (%) curve when seawater and water with 20 g/L Cl- were used as water sources in the leach solution. (b) Redox potential (mV) versus time (h) when seawater and water with 20 g/L Cl- were used as a source of water in the leach solution. Experimental conditions: [NaNO3] = [H2SO4] = 0.7 M, 45 °C and sample A.](https://d2c0zrx8qw0prh.cloudfront.net/images/Article_Images/ImageForArticle_1652_16481098462283659.png)
Figure 6. (a) Comparison of copper extraction (%) curve when seawater and water with 20 g/L Cl− were used as water sources in the leach solution. (b) Redox potential (mV) versus time (h) when seawater and water with 20 g/L Cl− were used as a source of water in the leach solution. Experimental conditions: [NaNO3] = [H2SO4] = 0.7 M, 45 °C and sample A. Image Credit: Hernández, et al., 2020
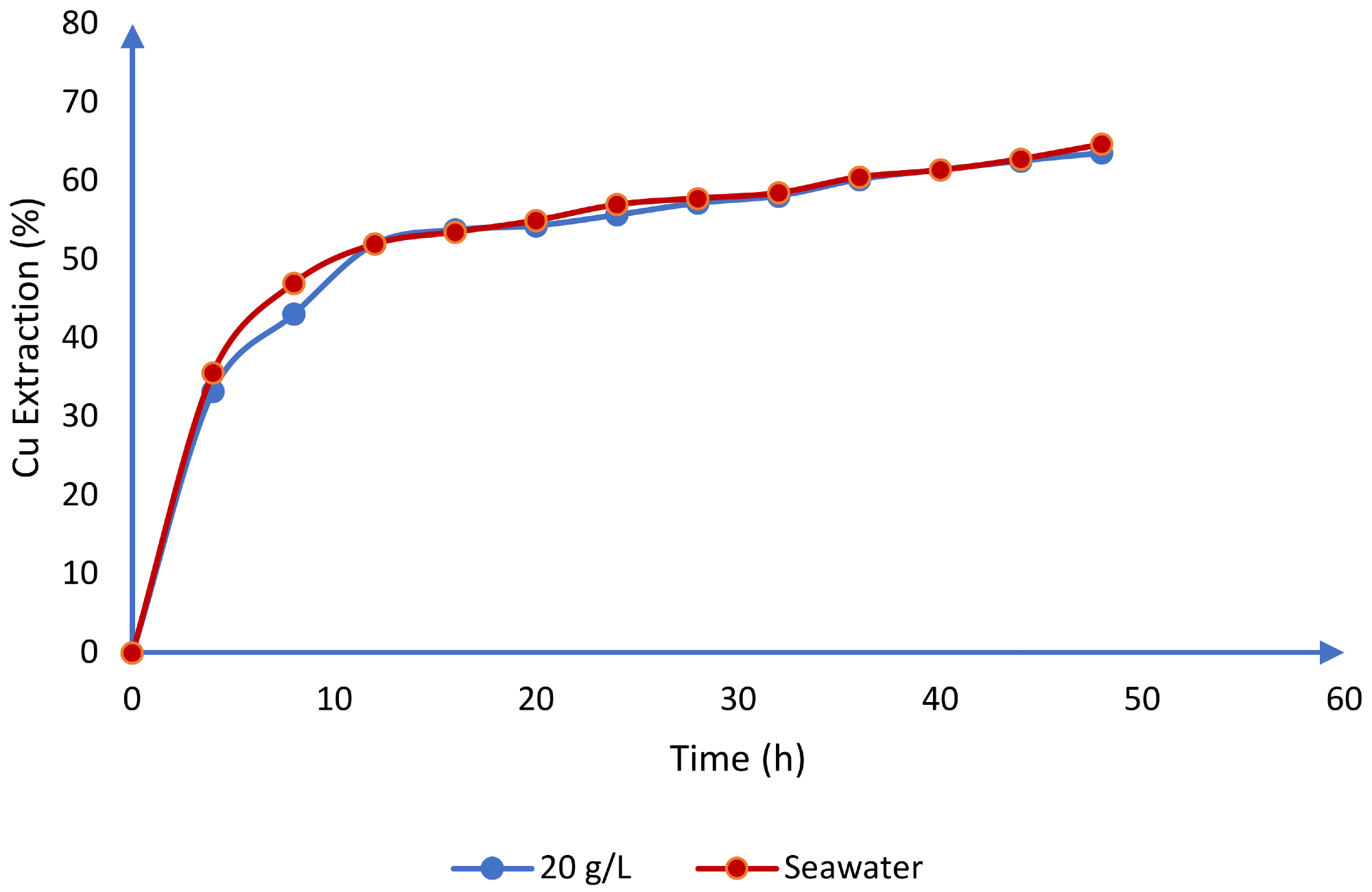
Figure 7. Comparison between the use of seawater and synthetic chloride (20 g/L) for the dissolution of chalcocite in an acid medium (T = 25 °C, H2SO4 = 0.5 mol/L). Image Credit: Toro, et al., 2019
There are now 14 water desalination facilities linked to mining operations (Table 3), with more planned in the future (Table 4).
Table 3. Land registry of desalination plants and seawater impulsion systems in copper mining in operation. Source: Cochilco, 2015
Mine |
Desalination Capacity (L/s) |
Seawater Use Capacity (L/s) |
Length of Water Transport Pipes (km) |
Michilla |
75 |
23 |
- |
Planta J.A. Moreno (Taltal) |
- |
15 |
- |
Las Cenizas (Taltal) |
9 |
12 |
7 |
Mantos de la Luna |
20 |
5 |
8 |
Pampa Camarones |
- |
25 |
12 |
Centinela |
50 |
1500 |
145 |
Cap Minería |
600 |
- |
120 |
Escondida (Planta Coloso) |
525 |
- |
180 |
Antucoya |
20 |
280 |
145 |
Candelaria |
300 |
- |
110 |
Mantoverde |
120 |
- |
42 |
Sierra Gorda |
- |
1315 |
142 |
Escondida EWS |
2500 |
- |
180 |
Candelaria |
700 |
- |
110 |
Table 4. Future projects of desalination plants and seawater impulsion systems in copper mining. Source: Altaley, 2021
Mine |
Desalination Capacity (L/s) |
Seawater Use capacity (L/s) |
Length of Water
Transport Pipes (km) |
Start-Up Year |
Los Pelambres |
400 |
- |
150 |
2021 |
Spence Growth Option |
800 (1600
potential) |
- |
154 |
2021 |
MantoVerde |
Add 260 to
current capacity |
- |
42 |
2022 |
ENAPAC (Múltiples mineras de la zona
norte de Chile) |
2630 |
- |
- |
2022 |
Quebrada Blanca |
850 (1200
potential) |
- |
160 |
2023 |
CODELCO (Chuquicamata,
Radomiro Tomic y
Ministro Hales) |
840 (1956
potential) |
- |
160 |
2023 |
Santo Domingo |
30 |
400 |
112 |
2024 |
COLLAHUASI |
525 (1050
potential) |
- |
195 |
2024 |
Diego de Almagro |
- |
315 |
61 |
2025 |
Centinela |
- |
1650 |
145 |
2025 |
El Abra |
500 |
- |
- |
2027 |
Nueva Unión |
700 |
- |
- |
2028 |
Desalination procedures produce brine, which is a waste product that is returned to the sea in vast quantities and has greater chemical element content (Table 5). Furthermore, brine is denser than seawater. As a result, it cannot combine with the sea and decants at the ocean’s bottom.
Table 5. Composition of wastewater from desalination plants used in previous studies. Source: Toro, et al., 2022
Previous Research |
[5] |
[57] |
Compound |
Concentration (g/L) |
Concentration (g/L) |
Fluoride (F−) |
0.002 |
0.002 |
Calcium (Ca2+) |
0.8 |
0.4 |
Magnesium (Mg2+) |
2.65 |
2.29 |
Sodium (Na+) |
20.85 |
19.77 |
Potassium (K+) |
0.82 |
0.75 |
Bicarbonate (HCO3−) |
1.1 |
0.236 |
Chloride (Cl−) |
39.16 |
36.07 |
Calcium carbonate (CaCO3) |
13 |
10 |
Table 6. Comparison between previous investigations for the dissolution of copper sulfide minerals in an acid medium using wastewater. Source: Toro, et al., 2022
Research |
Mineral |
Leaching Agent |
Parameters |
Cu Extraction (%) |
Reference |
Influence of seawater and discard brine on the dissolution of copper ore and copper concentrate |
Copper Oxides
(Atacamite and
Chrysocolla),
Chalcopyrite
Concentrate,
Chalcopyrite Mineral |
H2SO4, NaCl, Discard salts |
Chloride concentration,
cure time, potential
redox variation in the
leaching solution |
92 |
[49] |
Leaching of Pure Chalcocite in a Chloride Media Using Sea Water and Wastewater |
Chalcocite |
H2SO4,
NaCl |
Chloride concentration,
acid concentration |
67.75 |
[26] |
Leaching of Pure Chalcocite in a Chloride Media Using Wastewater at High Temperature |
Chalcocite |
H2SO4,
NaCl |
Acid concentration,
chloride concentration,
temperature |
97 |
[50] |
Caliche and Seawater, Sources of Nitrate and Chloride Ions to Chalcopyrite Leaching in Acid Media |
Chalcopyrite |
H2SO4, NaNO3, NaCl |
Temperature, solid/
liquid ratio, chloride
concentration, particle
size, nitrate
concentration, acid
concentration, cure
time |
92.3 |
[57] |
Dissolution of pure chalcopyrite with manganese nodules and wastewater |
Chalcopyrite |
H2SO4, NaCl,
MnO2 |
stirring speed, acid concentration, MnO2 concentration, potential variation in the leaching solution |
77 |
[5] |
Leaching Chalcopyrite with High MnO2 and Chloride Concentrations |
Chalcopyrite |
H2SO4, MnO2 |
Particle size, temperature |
71 |
[25] |
Use of Seawater/Brine and Caliche’s Salts as Clean and Environmentally Friendly Sources of Chloride and Nitrate Ions for Chalcopyrite Concentrate Leaching |
Chalcopyrite concentrate |
H2SO4, caliche
salts |
Acid concentration,
nitrate concentration,
chloride concentration,
temperature, cure time |
90.6 |
[56] |
Leaching of Pure Chalcocite with Reject Brine and MnO2 from Manganese Nodules |
Chalcocite |
H2SO4, NaCl,
MnO2 |
chloride concentration,
acid concentration,
potential redox
variation in the leaching
solution, MnO2
concentration |
71 |
[58] |
As a result, using saltwater or wastewater does not enhance oxidized mineral leaching. In contrast, the scientists found that while working with 100 days of curing and subsequent leaching in columns for a chalcopyrite mineral, wastewater (43%) outperforms saltwater (37%) in 80 days of irrigation in their trials.
Employing nitrate ions as an oxidizing agent, the authors examined the use of distilled water, salt water, and wastewater to leach chalcopyrite, with wastewater yielding the highest copper extractions (Figure 8).
![Copper extraction (%) versus time (h) when different dissolvents were used: distilled water (water), seawater, and brine. Experimental conditions: [NaNO3] = 0.7 M, [H2SO4] = 0.7 M, -150 + 75 µm, S/L ratio 2 g/L, 45 °C, 350 rpm, sample A.](https://d2c0zrx8qw0prh.cloudfront.net/images/Article_Images/ImageForArticle_1652_16481098566864703.png)
Figure 8. Copper extraction (%) versus time (h) when different dissolvents were used: distilled water (water), seawater, and brine. Experimental conditions: [NaNO3] = 0.7 M, [H2SO4] = 0.7 M, −150 + 75 µm, S/L ratio 2 g/L, 45 °C, 350 rpm, sample A. Image Credit: Hernández, et al., 2020
The authors claim that when working with high chloride concentrations, it is feasible to dissolve chalcopyrite at room temperature and avoid passivation (Figure 9). According to the researchers, it is possible to keep the voltage consistent between 580 and 650 mV using these operational conditions, resulting in 77% Cu extraction (Figure 10).
Furthermore, an examination of the residues collected revealed that no contaminating elements, such as stable elemental sulfur, were formed, and that other elements present, such as MgO and CaSO4, had no effect on the extraction of Cu (Figure 11).
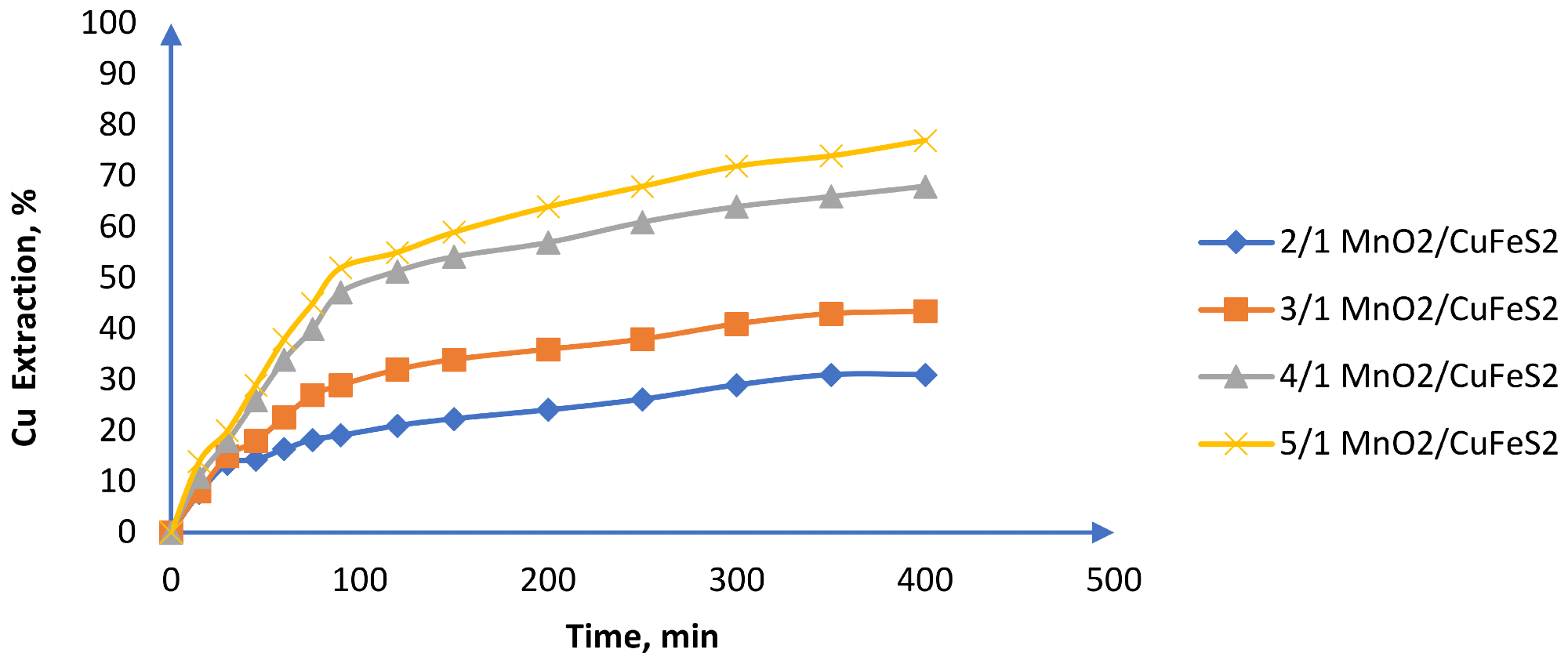
Figure 9. Effect of the MnO2/CuFeS2 ratio on Cu extraction (25 °C, CuFeS2 size: −47 + 38 m, MnO2 size: −75 + 53 m, 1 mol/L H2SO4 and 39.16 g/L of chloride). Image Credit: Toro, et al., 2019
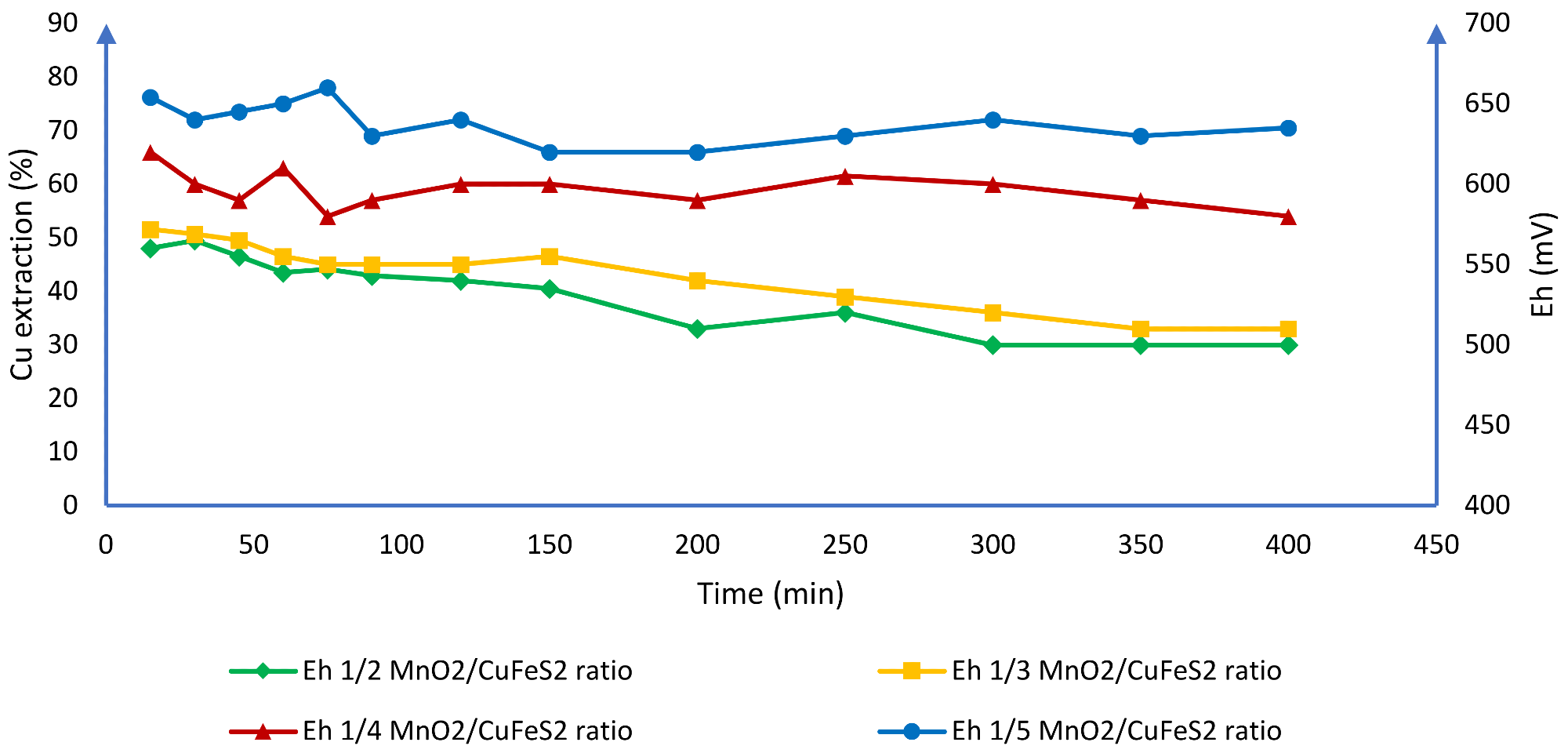
Figure 10. Effect of the potential in the solution of Cu at different ratios of MnO2/CuFeS2. Image Credit: Toro, et al., 2019
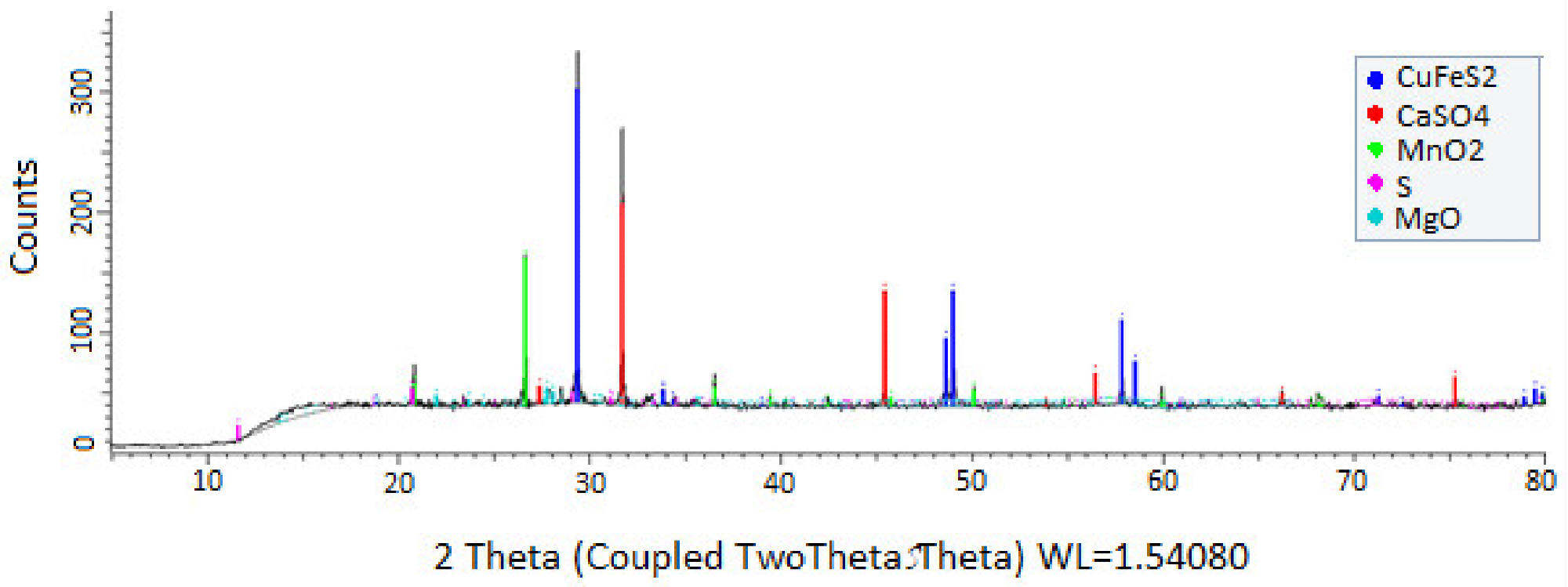
Figure 11. X-ray powder diffraction patterns for the residue mineral after being leached with wastewater at 25 °C in a time of 90 min. CuFeS2 size: −47 + 38 μm, MnO2 size: −75 + 53 μm, MnO2/CuFeS2 to 5/1 and 39.16 g/L chloride). Image Credit: Toro, et al., 2019
Finally, the researchers discovered that when chalcocite is leached in a chlorinated system, it dissolves rapidly (exponentially) until 50% of the Cu is extracted, which is attributable to the creation of covellite, which has slower dissolving kinetics.
The researchers found that adding MnO2 to the system at low concentrations enhances the extraction of Cu from Cu2S in a short period of time, which is relevant in continual leaching processes (Table 7).
Table 7. Comparison of chalcocite dissolution with reject brine and the effect of MnO2. Source: Toro, et al., 2022
Experimental Conditions and Results |
[26] |
[58] |
Temperature (°C) |
25 |
25 |
Particle size of Cu2S (um) |
−147 + 104 |
−147 + 104 |
H2SO4 concentration (mol/L) |
0.5 |
0.5 |
MnO2/Cu2S ratio (w/w) |
- |
0.25:1 |
Dissolution in seawater after 4 h (%) |
32.8 |
35.6 |
Dissolution in reject brine after 4 h (%) |
36 |
40 |
Dissolution in seawater after 48 h (%) |
63.4 |
64.7 |
Dissolution in reject brine after 48 h (%) |
64.6 |
66.2 |
Conclusion
There are many projects for desalination plants and systems of seawater impulsion to feed mining activities by the year 2030, depending on the behavior of saltwater projection in mining processes. The use of fresh water in major copper mining operations by government organizations is also restricted. As a result, it is reasonable to predict that the use of seawater and wastewater in mining will continue to expand in the coming years.
On the other hand, wastewater from desalination plants has shown good outcomes for the dissolution of copper from sulfide minerals in recent research, which is due to the fact that it has a higher concentration of chloride ions than seawater (roughly double), allowing the system to operate at higher redox potential values.
As a result, it can be stated that wastewater from water desalination plants can be used in heap leaching procedures for copper sulfide minerals as long as the expense of transporting the wastewater from the desalination plants to the mining locations can be sustained.
Journal Reference:
Toro, N., Gálvez, E., Robles, P., Castillo, J., Villca, G., Salinas-Rodríguez, E. (2022) Use of Alternative Water Resources in Copper Leaching Processes in Chilean Mining Industry—A Review. Metals, 12(3), p. 445. Available Online: https://www.mdpi.com/2075-4701/12/3/445/htm.
References and Further Reading
- Hossain, M Z (2015) Water: The Most Precious Resource of Our Life. Global Journal of Advamced Research, 2(9), pp. 1436–1445. Available at: http://www.gjar.org/articles/WATER--THE-MOST-PRECIOUS-RESOURCE-OF-OUR-LIFE
- Kirk Cochran, J (2014) Oceanography. In: Reference Module in Earth Systems and Environmental Sciences; Elsevier: Amsterdam, The Netherlands, 142, pp. 418–421. ISBN 9780124095489.
- Torres Albornoz, DA. (2021) Copper and Manganese Extraction Through Leaching Processes; Universidad Politécnica de Cartagena: Cartagena, Spain.
- Cisternas, L A & Gálvez, E D (2018) The use of seawater in mining. Mineral Processing and Extractive Metallurgy Review, 39(1), pp. 18–33. doi.org/10.1080/08827508.2017.1389729.
- Toro, N., et al. (2019) Dissolution of pure chalcopyrite with manganese nodules and waste water. Journal of Materials Research and Technology, 9(1), pp. 798–805. doi.org/10.1016/j.jmrt.2019.11.020.
- Filho, W. L., et al. (2020) Responsible Consumption and Production; Encyclopedia of the UN Sustainable Development Goals; Springer International Publishing: Cham, Switzerland; ISBN 978-3-319-95725-8. doi.org/10.1007/978-3-319-95726-5.
- Valavanidis, A (2019) “Blue Planet” Is Expected to Experience Severe Water Shortages? Available at: http://chem-tox-ecotox.org/blue-planet-is-expected-to-experience-severe-water-shortages-how-climate-change-and-rising-temperatures-are-threatening-the-global-water-cycle-on-earth/.
- Halmaghi, E E & Moşteanu, D (2019) Considerations on Sustainable Water Resources Management. International conference KNOWLEDGE-BASED ORGANIZATION, 25(1), pp. 236–240. doi.org/10.2478/kbo-2019-0038.
- Linton, J & Budds, J (2014) The hydrosocial cycle: Defining and mobilizing a relational-dialectical approach to water. Geoforum, 57, pp. 170–180. doi.org/10.1016/j.geoforum.2013.10.008.
- Ramanathan, V., et al. (2001) Aerosols, climate and the hydrological cycle. Science, 294(5549), pp. 2119–2124. doi.org/10.1126/science.1064034.
- Kamojjala, S. (2018) World Environmental and Water Resources Congress 2018: Hydraulics and Waterways, Water Distribution Systems Analysis, and Smart Water. In: American Society of Civil Engineers, Reston, VA, USA, p. ISBN 9780784481424. doi.org/10.1061/9780784481424.fm.
- Balasubramanian, A. (2015) The World’ S Water Crisis. Research Gate, 44, pp. 1–14.
- Alsharhan, A S & Rizk, Z E (2020) Water Resources and Integrated Management of the United Arab Emirates. In: Springer Nature: Berlin/Heidelberg, Germany. p. ISBN 9783030316839. Available at: https://link.springer.com/book/10.1007/978-3-030-31684-6.
- (2019) Mch Gestión del Agua, un Reto País. Available at: https://www.mch.cl/editorial/gestion-del-agua-un-reto-pais/#.
- Brenner, A. (2011) Limitations and Challenges of Wastewater Reuse in Israel. Clean Soil and Safe Water. pp. 3–9. Available at: https://link.springer.com/chapter/10.1007/978-94-007-2240-8_1
- Friedler, E (2001) Water reuse—An integral part of water resources management: Israel as a case study. Water Policy, 3(1), pp. 29–39. doi.org/10.1016/S1366-7017(01)00003-4.
- Kfir, O., et al. (2012) The effect of reservoir operational features on recycled wastewater quality. Resources, Conservation and Recycling, 68, pp. 76–87. doi.org/10.1016/j.resconrec.2012.08.002.
- Cameira, M d R & Pereira, L S (2019) Innovation Issues in Water, Agriculture and Food. Water, 11(6), p. 1230. doi.org/10.3390/w11061230.
- Kumar, A & Goyal, K (2020) Water reuse in India: Current perspective and future potential. Advances in Chemical Pollution, Environmental Management and Protection, 6, pp. 33–63. doi.org/10.1016/bs.apmp.2020.07.011.
- Pereira, L S (2017) Water, Agriculture and Food: Challenges and Issues. Water Resources Management, 31, pp. 2985–2999. doi.org/10.1007/s11269-017-1664-z.
- Tariq, M. A. U. R., et al. (2020) Identification of Major Inefficient Water Consumption Areas Considering Water Consumption, Efficiencies, and Footprints in Australia. Applied Sciences, 10(18), p. 6156. doi.org/10.3390/app10186156.
- Aitken, D., et al. (2016) Water Scarcity and the Impact of the Mining and Agricultural Sectors in Chile. Sustainability, 8(2), p. 128. doi.org/10.3390/su8020128.
- Jerez, B., et al. (2021) Lithium extractivism and water injustices in the Salar de Atacama, Chile: The colonial shadow of green electromobility. Political Geography, 87, p. 102382. doi.org/10.1016/j.polgeo.2021.102382.
- Liu, W., et al. (2019) Spatiotemporal patterns of lithium mining and environmental degradation in the Atacama Salt Flat, Chile. International Journal of Applied Earth Observation and Geoinformation, 80, pp. 145–156. doi.org/10.1016/j.jag.2019.04.016.
- Torres, D., et al. (2020) Leaching Chalcopyrite with High MnO2 and Chloride Concentrations. Metals, 10(1), p. 107. doi.org/10.3390/met10010107.
- Toro, N., et al. (2019) Leaching of pure chalcocite in a chloride media using sea water and waste water. Metals, 9(7), p. 780. doi.org/10.3390/met9070780.
- Tundisi, J G (2008) Water resources in the future: Problems and solutions. Estudos Avançados, 22(63), pp. 7–16. doi.org/10.1590/S0103-40142008000200002.
- Herrera-León, S., et al. (2019) Current situation and major challenges of desalination in Chile. Desalination and Water Treatment, 171, pp. 93–104. Available at: https://www.deswater.com/DWT_abstracts/vol_171/171_2019_93.pdf.
- Peters, N E & Meybeck, M (2000) Water quality degradation effects on freshwater availability: Impacts of human activities. Water Internatinal, 25(2), pp. 185–193. doi.org/10.1080/02508060008686817.
- Ridoutt, B G & Pfister, S (2010) A revised approach to water footprinting to make transparent the impacts of consumption and production on global freshwater scarcity. Global Environmental Change, 20(1), pp. 113–120. doi.org/10.1016/j.gloenvcha.2009.08.003.
- (2019) COCHILCO. In: Consumo de Agua en la Minería del Cobre; COCHILCO: Santiago, Chile.
- Mudd, G.M. (2009) In: The Sustainability of Mining in Australia: Key Production Trends and Their Environmental Implications for the Future; Department of Civil Engineering, Monash University: Melbourne, Australia, 2009; ISBN 9780980319941. Available at: https://www.researchgate.net/publication/313050247_The_sustainability_of_mining_in_Australia_key_production_trends_and_their_environmental_implications_for_the_future
- Toro, N., et al. (2020) Seabed mineral resources, an alternative for the future of renewable energy: A critical review. Ore Geology Reviews, 126, p. 103699. doi.org/10.1016/j.oregeorev.2020.103699.
- Pérez, K., et al. (2020) Statistical Study for Leaching of Covellite in a Chloride Media. Metals, 10(4), p. 477. doi.org/10.3390/met10040477.
- Wisskirchen, C W J & Vásquez, F (2012) In Considerations for seawater in mining: Approaches to evaluate ARD and metals leaching potential. In: Proceedings of the 3rd International Congress on Water Management in the Mining Industry, Santiago, Chile, 6–8 June 2012, pp. 524–534.
- Herrera, L (2008) Problemas Operativos Asociados al Desarrollo de Algas y Cianobacterias en Sistemas de Agua en Minería: Uso de Agua de Mar en Procesos Industriales; Xpert, Ingeniería y Producción S.A.: Santiago, Chile.
- Busch, M & Mickols, W E (2004) Reducing energy consumption in seawater desalination. Desalination, 165, pp. 299–312. doi.org/10.1016/j.desal.2004.06.035.
- Calabrò, V & Basile, A (2011) Economic analysis of membrane use in industrial applications. Advanced Membrane Science and Technology for Sustainable Energy and Environmental Applications, pp. 90–109. doi.org/10.1533/9780857093790.1.90.
- Feria-Díaz, J. J., et al. (2021) Recent Desalination Technologies by Hybridization and Integration with Reverse Osmosis: A Review. Water, 13(10), p. 1369. doi.org/10.3390/w13101369.
- Jones, E., et al. (2019) The state of desalination and brine production: A global outlook. Science of The Total Environment, 657, pp. 1343–1356. doi.org/10.1016/j.scitotenv.2018.12.076.
- Cisternas, L & Moreno, L (2014) Seawater in Mining. Fundamentals and Applications. Technological information, 25(4), pp. 1–2. doi.org/10.4067/S0718-07642014000400001.
- Toro Villarroel, N R (2020) Optimización de Parámetros Para la Extracción de Elementos Desde Minerales en Medios Ácido; Universidad Politécnica de Cartagena: Cartagena, Spain, 2020. doi.org/10.31428/10317/8504.
- Hernández, PC (2013) Estudio Del Equilibrio Sólido-Líquido De Sistemas Acuosos De Minerales De Cobre Con Agua De Mar, Aplicado A Procesos De Lixiviación; Universidad De Antofagasta: Antofagasta, Chile.
- Castellón, C.I.; et al. (2012) . An alternative process for leaching chalcopyrite concentrate in nitrate-acid-seawater media with oxidant recovery. Metals, 10(4), p. 518. doi.org/10.3390/met10040518.
- Cerda, C. P., et al. (2018) Effect of pretreatment on leaching primary copper sulfide in acid-chloride media. Minerals, 8(1), p. 1. doi.org/10.3390/min8010001.
- Hernández, P. C., et al. (2018) Leaching of chalcopyrite in acidified nitrate using seawater-based media. Minerals, 8(6), p. 238. doi.org/10.3390/min8060238.
- Hernández, P. C., et al. (2019) Accelerating copper leaching from sulfide ores in acid-nitrate-chloride media using agglomeration and curing as pretreatment. Minerals, 9(4), p. 250. doi.org/10.3390/min9040250.
- Velásquez-Yévenes, L., et al. (2018) Leaching of chalcopyrite ore agglomerated with high chloride concentration and high curing periods. Hydrometallurgy, 181, pp. 215–220. doi.org/10.1016/j.hydromet.2018.10.004.
- Velásquez-Yévenes, L & Quezada-Reyes, V (2018) Influence of seawater and discard brine on the dissolution of copper ore and copper concentrate. Hydrometallurgy, 180, pp. 88–95. doi.org/10.1016/j.hydromet.2018.07.009.
- Pérez, K., et al. (2020) Leaching of pure chalcocite in a chloride media using waste water at high temperature. Metals, 10(3), p. 384. doi.org/10.3390/met10030384.
- Saldaña, M., et al. (2020) Development of an empirical model for copper extraction from chalcocite in chloride media. Hemijska industrija, 74(5), pp. 285–292. doi.org/10.2298/HEMIND200424031S.
- Millero, F. J., et al. (2008) The composition of Standard Seawater and the definition of the Reference-Composition Salinity Scale. Deep Sea Research Part I: Oceanographic Research Papers, 55(1), pp. 50–72. doi.org/10.1016/j.dsr.2007.10.001.
- Fisher, W.W., et al. (1992) Comparison of chalcocite dissolution in the oxygenated, aqueous sulfate and chloride systems. Mineraks Engineering, 5(7), pp. 817–834. doi.org/10.1016/0892-6875(92)90248-8.
- Hashemzadeh, M., et al. (2019) Modelling the kinetics of chalcocite leaching in acidified cupric chloride media under fully controlled pH and potential. Hydrometallurgy, 189, p. 105114. doi.org/10.1016/j.hydromet.2019.105114.
- Senanayake, G. (2007) Chloride assisted leaching of chalcocite by oxygenated sulphuric acid via Cu(II)-OH-Cl. Minerals Engineering, 20(11), pp. 1075–1088. doi.org/10.1016/j.mineng.2007.04.002.
- Hernández, P., et al. (2020) Use of Seawater/Brine and Caliche’s Salts as Clean and Environmentally Friendly Sources of Chloride and Nitrate Ions for Chalcopyrite Concentrate Leaching. Minerals, 10(5), p. 477. doi.org/10.3390/min10050477.
- Hernández, P., et al. (2020) Caliche and Seawater, Sources of Nitrate and Chloride Ions to Chalcopyrite Leaching in Acid Media. Metals, 10(5), p. 551. doi.org/10.3390/met10040551.
- Torres, D., et al. (2020) Leaching of Pure Chalcocite with Reject Brine and MnO2 from Manganese Nodules. Metals, 10(11), p. 1426. doi.org/10.3390/met10111426.
- (2020) COCHILCO. Ind: Proyeccion Agua Mineria del Cobre, 2019–2030; COCHILCO: Santiago, Chile.
- (2021) ALTALEY.In: MINERÍA VERDE: Oportunidades y Desafíos; ALTALEY: Vancouver, BC, Canada.
- Binici, A & Kaya, G K (2018) Effect of brine and dry salting methods on the physicochemical and microbial quality of chub (Squalius cephalus Linnaeus, 1758). Food Science and Technology, 38(Suppl. 1), pp. 66–70. doi.org/10.1590/1678-457X.15717.
- Sasakura Engineering Co., Ltd. (2021) Effective Utilization of Seawater—Fresh Water Generator and Seawater Desalination. Marine Engineering, 56(2), pp. 222–226. doi.org/10.5988/jime.56.222.
- Tan, G., et al. (2021) Ultrahigh and Stable Water Recovery of Reverse Osmosis-Concentrated Seawater with Membrane Distillation by Synchronously Optimizing Membrane Interfaces and Seawater Ingredients. ACS ES&T Water, 1(7), pp. 1577–1586. doi.org/10.1021/acsestwater.1c00087.
- Zhang, X., et al. (2021) A review of resource recovery from seawater desalination brine. Reviews in Environmental Science and Bio/Technology, 20, pp. 333–361. doi.org/10.1007/s11157-021-09570-4.
- Gude, V G (2015) Energy storage for desalination processes powered by renewable energy and waste heat sources. Applied Energy, 137, pp. 877–898. doi.org/10.1016/j.apenergy.2014.06.061.
- Bogdanović, G. D., et al. (2020) Chalcopyrite leaching in acid media: A review. Metallurgical and Materials Engineering, 26(2), pp. 177–198. doi.org/10.30544/526.
- Miki, H., et al. (2011) The kinetics of dissolution of synthetic covellite, chalcocite and digenite in dilute chloride solutions at ambient temperatures. Hydrometallurgy, 105(3–4), pp. 321–327. doi.org/10.1016/j.hydromet.2010.11.004.
- Ruiz, M.C., et al. (2015) Galvanic Effect of Pyrite on Chalcopyrite Leaching in Sulfate-Chloride Media. Mineral Processing and Extractive Metallurgy Review, 36(1), pp. 65–70. doi.org/10.1080/08827508.2013.868349.
- Sokić, M., et al. (2019) Kinetics of Chalcopyrite Leaching by Hydrogen Peroxide in Sulfuric Acid. Metals, 9(11), p. 1173. https://doi.org/10.3390/met9111173.