Seismic noise can be generated through continuous vibrations at different frequencies inside the earth. Typically, there are three common seismic noise sources encountered in the field: natural seismic noise, man-made electromagnetic interference (EMI), and man-made seismic noise. This noise can monitor a mine’s seismicity and structural integrity to detect potential ground hazards.
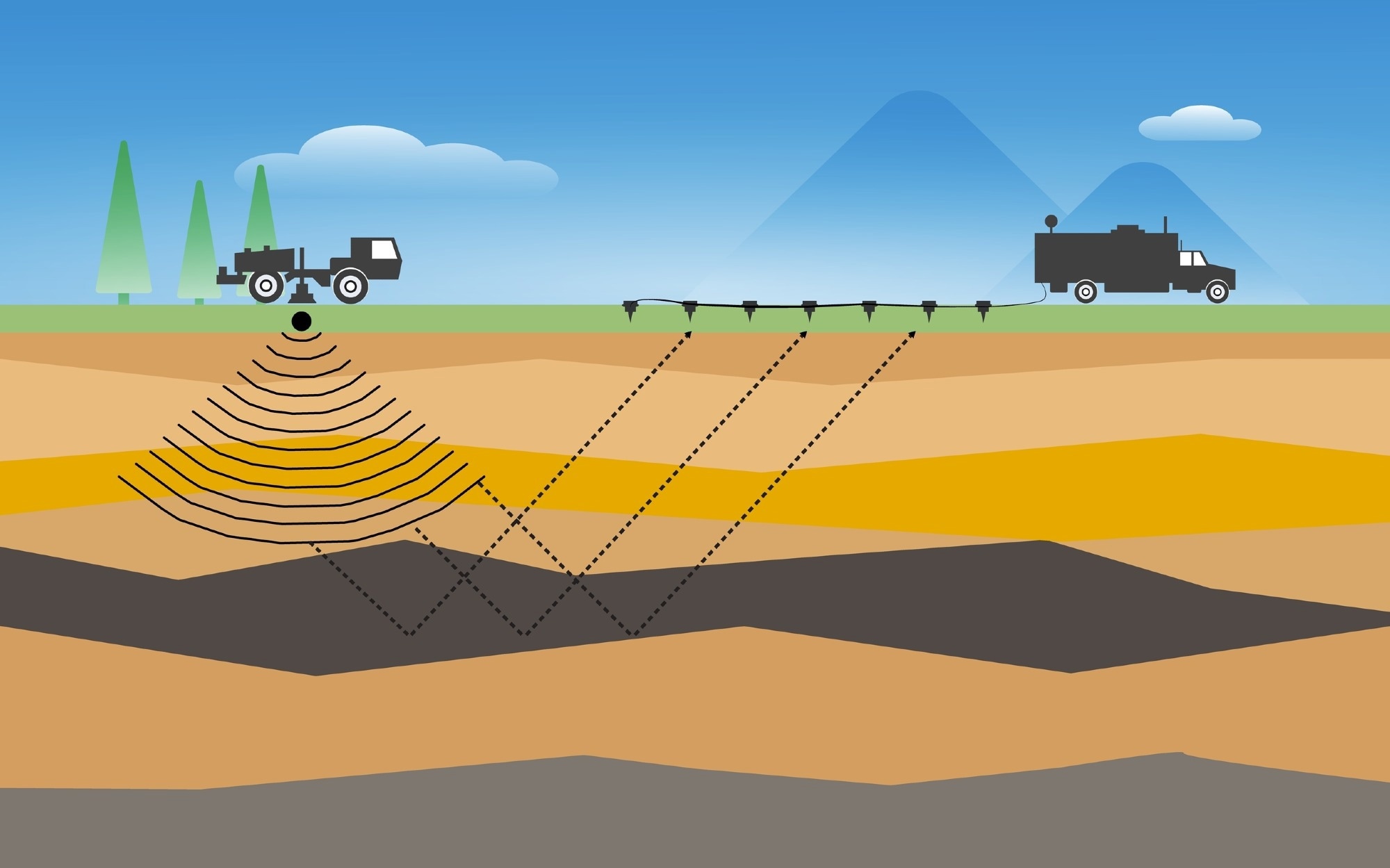
Image Credit: calcassa/Shutterstock.com
Potential Hazards in Mining
The mining process is non-spontaneous and can induce stresses in the mining region. On average, the mining-induced deformation rate is greater than the average slip rate of tectonic plates by a magnitude of at least two orders.
Deformation and stress-relaxation caused by underground openings are followed by rock extractions, which initiate bulk seismic activity in the mines.
During stress-relaxation, the accumulated potential energy in the rock mass may be released suddenly or gradually.
A sudden inelastic deformation will lead to a seismic event.
The subsequent seismic waves vary in amplitude and frequency depending on the rock’s strength and stress state, the seismic source’s size, and the magnitude of the deformation rate during fracture.
The seismic response of rocks in mines can be monitored to quantify, prevent, control, and even predict potential rock mass destabilization resulting in rock bursts. Furthermore, blasting vibrations, energy release due to rock crack propagation, and mechanical vibrations induce microseismic events in underground mines.
Increased mining-induced stress can also cause the filling up of cracks, fractures, pores, and voids, which increases the elastic wave velocity.
Microseismic events of varying magnitudes due to rock fracturing can excite elastic waves in the surrounding rocks.
Although these events are of smaller magnitudes, their locations are superficial and closer to the surface. Therefore, they can inflict major damage and loss of life and have a high occurrence rate.
Currently Used Methods to Monitor the Mining Hazards
Although past data can be beneficial for comparing seismic rock masses to various mining scenarios, it is not the optimum indicator of future hazards due to the irregular nature of mass loading. Seismic monitoring is widely used to detect, locate, and estimate the size or strength of ground movements.
Seismicity recordings in the mines of the United States ranged from using small-scale arrays, collecting analog signals by geophones, and personal computer seismic unified data structures (PC-SUDS).
In Germany, seismicity monitoring at underground coal mines is conducted by 60 seismic stations, which use three-component 4.5-Hz geophones operating at 500 samples/second. About 20 mines in Poland use the seismic network developed by the central mining institute (CMI) comprising conventional and broadband 1-Hz short-period triaxial sensors.
In Australia, fracturing processes due to longwall caving have been delineated using microseismic monitoring systems developed from numerous temporary research networks. Most of these networks utilized a triaxial geophone sensor. These sensors are grouted into the drilled surface boreholes that provide real-time analysis of the microseismic data.
Methods such as ambient noise tomography record dispersive surface waves and create a three-dimensional (3D) model of the s-wave velocity structure. These passive methods provide a low-cost solution to image the subsurface, delineate structural integrity, and help avoid potential hazards.
Techniques such as velocity tomography use seismic waves produced from mining-induced microseismic activities such as explosions to structure the stress state or the rock mass conditions. However, the quality of results from velocity tomography can be affected by factors such as accurate arrival time picking, high-resolution source location, ray tracing, and efficient inversion algorithms.
Velocity tomography is primarily used to monitor rock mass stability, detect geological structure and discontinuities, and prevent rock burst hazards.
Recent Research and its Future in Mining
A recent study by Wu and team evaluated noise cross-correlations functions (CCFs) by analyzing one month of surface 17 geophone array data at a longwall coal mine. Their work established that the CCFs related to the ambient noise could be isolated and used to trace mining-induced signals, enabling further research on structural integrity.
Other recent studies have reported using passive seismic data to model the subsurface as an attractive approach for implementing mine safety and preventing hazards.
Seismic noise tomography is another environmentally friendly, inexpensive technique used in exploration and subsurface structure detection. This, coupled with the latest advancements in compact autonomous seismic recording stations and low-cost data collection spanning over a month, makes it an attractive prospect in future mining applications.
Methods such as velocity tomography are advantageous due to their broad monitoring range, allowing them to be utilized at a mine scale or a regional scale and carry out long-term continuous observations. However, it is limited due to deviations of the calculated source locations from their actual positions and can be less reliable in mined-out areas with lesser ray coverage.
Velocity tomography has efficiently analyzed mining-induced stress distribution in hard rock and underground coal mines. Hence, this method’s ability to assess rock instability and rock disturbances can be helpful in early predicting and evaluating mining-induced hazards and enhanced ground control. However, the results of this method are not always effective and successful and hence require improvements in timeliness and accuracy.
References and Further Reading
Swanson P, Boltz MS, and Chambers D. Spokane, Seismic monitoring strategies for deep longwall coal mines. WA: U.S. Department of Health and Human Services, Centers for Disease Control and Prevention, National Institute for Occupational Safety and Health, DHHS (NIOSH) Publication No. 2017-102, RI 9700. https://www.cdc.gov/niosh/mining/works/coversheet1944.html
Mendecki, Aleksander & Lotter, Ernest. (2011). Modelling Seismic Hazard for Mines. https://www.researchgate.net/publication/236183155_Modelling_Seismic_Hazard_for_Mines
Mendecki, Aleksander & Van Aswegen, Gerrie &Mountfort, Peter. (1999). A guide to routine seismic monitoring in mines. https://www.researchgate.net/publication/236204233_A_guide_to_routine_seismic_monitoring_in_mines
Durkin, John, Greenfield, Roy J., Evaluation of the Seismic System for Locating Trapped Miners, Buereau of Mines Report of Investigations/1981, United States Department of the Interior. https://www.cdc.gov/niosh/mining/UserFiles/works/pdfs/ri8567.pdf
Nicola Ramm, Tjaart de Wit & Gerrit Olivier (2019) Passive Seismic Imaging for Mineral Exploration, ASEG Extended Abstracts, 2019:1, 1-3, DOI: 10.1080/22020586.2019.12073177. https://doi.org/10.1080/22020586.2019.12073177
Li, Y.; Deng, H.; Wen, L.; Qin, Y.; Xu, X. Method for Identifying and Forecasting Mining-Induced Earthquakes Based on Spatiotemporal Characteristics of Microseismic Activities in Fankou Lead/Zinc Mine. Minerals 2022, 12, 318. https://doi.org/10.3390/min12030318
Zhu, Qiankun& Zhao, Xingdong& Westman, Erik. (2021). Review of the Evolution of Mining-Induced Stress and the Failure Characteristics of Surrounding Rock Based on Microseismic Tomography. Shock and Vibration. 2021. 1-19. 10.1155/2021/2154857. https://www.researchgate.net/publication/354647108_Review_of_the_Evolution_of_Mining-Induced_Stress_and_the_Failure_Characteristics_of_Surrounding_Rock_Based_on_Microseismic_Tomography.
Santiago Rabade, Sin‐Mei Wu, Fan‐Chi Lin, Derrick J. A. Chambers; Isolating and Tracking Noise Sources across an Active Longwall Mine Using Seismic Interferometry. Bulletin of the Seismological Society of America 2022; doi: https://doi.org/10.1785/0120220031
Disclaimer: The views expressed here are those of the author expressed in their private capacity and do not necessarily represent the views of AZoM.com Limited T/A AZoNetwork the owner and operator of this website. This disclaimer forms part of the Terms and conditions of use of this website.